Breathing Beyond Earth: A Reliable Oxygen Production Architecture for Human Space Exploration
Alvaro Romero-CalvoGeorgia Tech Research Corporation The reliable and efficient operation of spacecraft life support systems is challenged in microgravity by the near absence of buoyancy. This impacts the electrolytic production of oxygen and hydrogen from water by forcing the adoption of complex multiphase flow management technologies. Still, water splitting plays an essential role in human […]
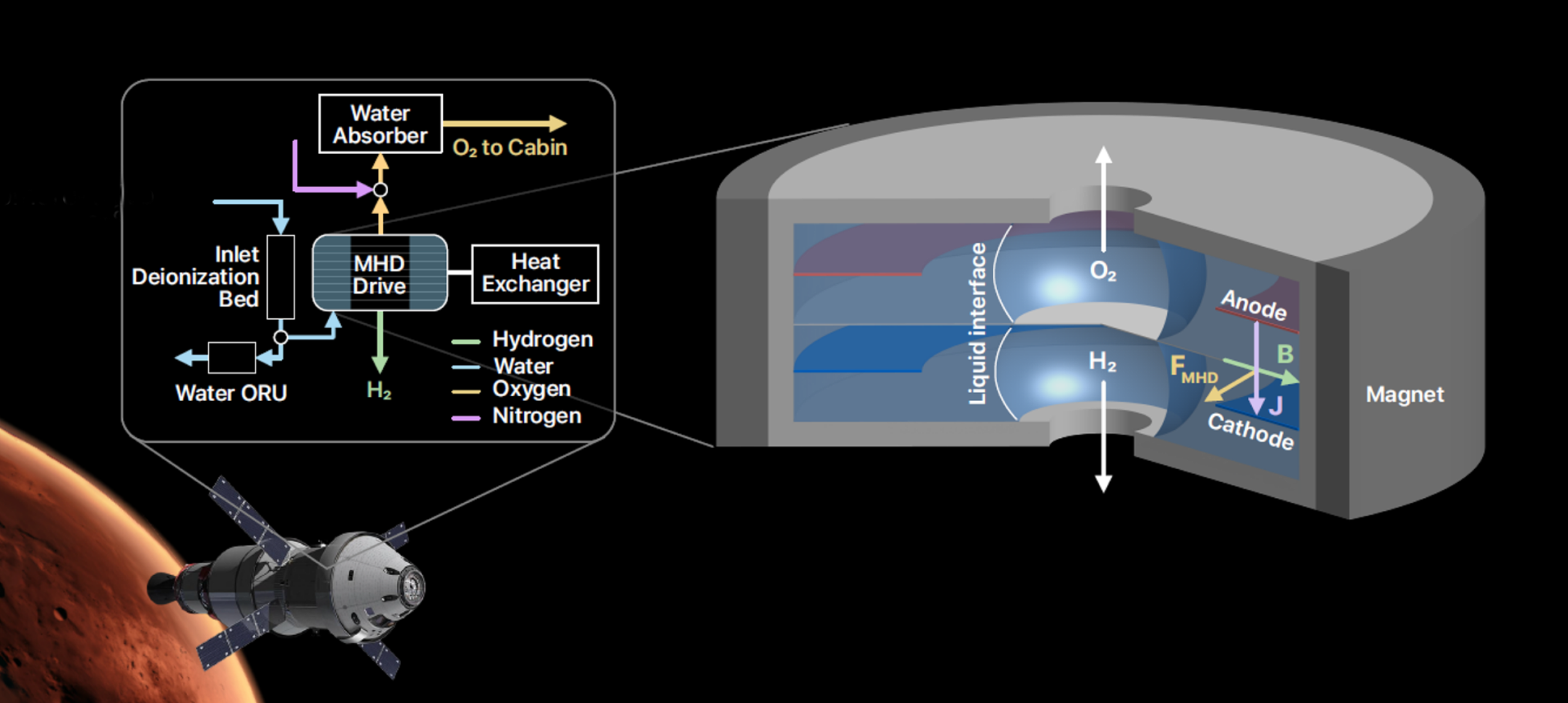
3 min read
Preparations for Next Moonwalk Simulations Underway (and Underwater)
Alvaro Romero-Calvo
Georgia Tech Research Corporation
The reliable and efficient operation of spacecraft life support systems is challenged in microgravity by the near absence of buoyancy. This impacts the electrolytic production of oxygen and hydrogen from water by forcing the adoption of complex multiphase flow management technologies. Still, water splitting plays an essential role in human spaceflight, closing the regenerative environmental control and life support loop and connecting the water and atmosphere management subsystems. Existing oxygen generation systems, although successful for short-term crewed missions, lack the reliability and efficiency required for long-duration spaceflight and, in particular, for Mars exploration.
During our Phase I NIAC effort, we demonstrated the basic feasibility of a novel water-splitting architecture that leverages contactless magnetohydrodynamic (MHD) forces to produce and separate oxygen and hydrogen gas bubbles in microgravity. The system, known as the Magnetohydrodynamic Oxygen Generation Assembly (MOGA), avoids the use of forced water recirculation loops or moving parts such as pumps or centrifuges for phase separation. This fundamental paradigm shift results in multiple operational advantages with respect to the state-of-the-art: increased robustness to over- and under-voltages in the cell stack, minimal risk of electrolyte leaching, wider operational temperature and humidity levels, simpler transient operation, increased material durability, enhanced system stability during dormant periods, modest water purity requirements, reduced microbial growth, and better component-level swap-ability, all of which result in an exceptionally robust system. Overall, these architectural features lead to a 32.9% mass reduction and 20.4% astronaut maintenance time savings with respect to the Oxygen Generation Assembly at the ISS for a four-crew Mars transfer, making the system ideally suited for long-duration missions. In Phase II, we seek to answer some of the key remaining unknowns surrounding this architecture, particularly regarding (i) the long-term electrochemical and multiphase flow behavior of the system in microgravity and its impact on power consumption and liquid interface stability, (ii) the transient operational modes of the MHD drive during start-up, shutdown, and dormancy, and (iii) architectural improvements for manufacturability and ease of repair. Toward that end, we will leverage our combined expertise in microgravity research by partnering with the ZARM Institute in Bremen and the German Aerospace Center to fly, free of charge to NASA, a large-scale magnetohydrodynamic drive system and demonstrate critical processes and components. An external review board composed of industry experts will assess the evolution of the project and inform commercial infusion. This effort will result in a TRL-4 system that will also benefit additional technologies of interest to NASA and the general public, such as water-based SmallSat propulsion and in-situ resource utilization.
Share
Details
Related Terms
What's Your Reaction?
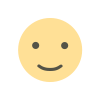
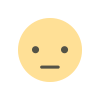
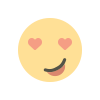
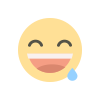
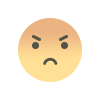
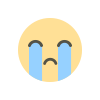
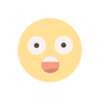